Quantum entanglement, often described as “spooky action at a distance,” represents one of the most intriguing phenomena in quantum physics. This phenomenon involves particles becoming interconnected in such a way that the state of one instantly influences the state of another, regardless of the distance separating them. First theorized by Albert Einstein, Niels Bohr, and others in the early 20th century, quantum entanglement challenges our classical understanding of physics. Through groundbreaking experiments and theoretical developments, scientists have uncovered its profound implications for technology, information theory, and computing. This article delves into the history, key experiments, applications, and future prospects of quantum entanglement, while also exploring its ethical and phil
Join tirfblog.com as we delve deeper into this topic.
1. Introduction to Quantum Entanglement
Quantum entanglement, a fundamental principle of quantum mechanics, unveils a deeply perplexing and counterintuitive aspect of the microscopic realm. Its essence lies in the interconnectedness of particles, where pairs or groups become linked in a way that the state of one particle directly influences the state of another, irrespective of the distance separating them. This instantaneous connection, famously dubbed “spooky action at a distance” by Einstein, defies classical notions of space and time, leaving us with a profound mystery.
The idea of entanglement was first proposed in the early 20th century by physicists like Albert Einstein, Niels Bohr, and Erwin Schrödinger. They struggled to understand the implications of this phenomenon, which seemed to defy the basic principles of locality and realism. Einstein, in particular, was doubtful, calling it “spooky action at a distance.” However, despite his reservations, numerous experiments have since confirmed entanglement, proving its vital role in quantum theory.
Quantum entanglement, far from being a mere theoretical concept, holds profound implications for our understanding of the universe. It has paved the way for groundbreaking technological advancements, including quantum computing and secure communication systems. As we continue to explore the intricacies of this captivating phenomenon, we uncover its scientific significance and its potential to revolutionize our world.
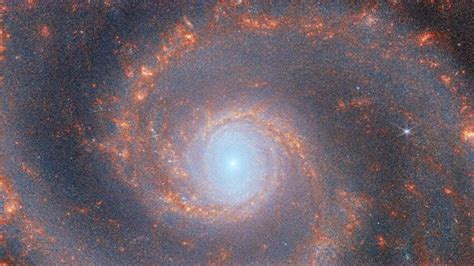
2. Historical Development and Theoretical Foundations
The historical development of quantum entanglement traces its roots back to the early 20th century, with seminal contributions from pioneers like Albert Einstein, Niels Bohr, and Erwin Schrödinger. In 1935, Einstein, Podolsky, and Rosen published their groundbreaking EPR paper, which challenged the completeness of quantum mechanics by introducing the concept of entanglement. Their argument revolved around the notion that if quantum mechanics were indeed complete, it would necessarily imply the existence of “spooky action at a distance.”
Schrödinger later coined the term “entanglement” to describe this phenomenon. Despite Einstein’s skepticism, Bohr defended the theory, asserting that entanglement was a natural consequence of quantum mechanics. Over time, theoretical advancements and experiments have confirmed the validity of entanglement. Notable developments include Bell’s theorem in the 1960s, which provided a way to test entanglement experimentally, and subsequent experiments that have repeatedly validated these predictions. These historical milestones laid the groundwork for understanding and exploring quantum entanglement in modern physics.
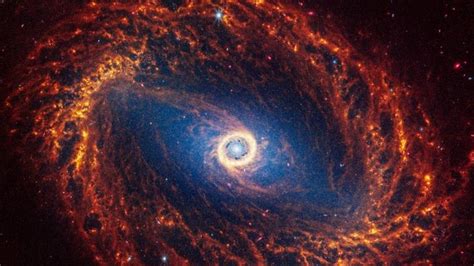
3. Key Experiments in Quantum Entanglement
Key experiments have been instrumental in validating quantum entanglement and expanding our comprehension of its implications. A landmark example is Alain Aspect’s 1982 experiment, which tested Bell’s theorem. Aspect’s team utilized entangled photon pairs to showcase that measurements performed on one photon instantaneously influenced the other, irrespective of their separation. This conclusively demonstrated the superiority of quantum mechanics over classical physics in explaining such phenomena.
John Clauser and Stuart Freedman’s experiments in the 1970s provided crucial initial experimental support for Bell’s inequalities. Technological advancements have since enabled increasingly sophisticated experiments, such as those involving entangled particles in quantum networks and quantum communication. These experiments not only validate the phenomenon of entanglement but also explore its potential applications in quantum computing and cryptography, further underscoring the profound implications of entanglement.
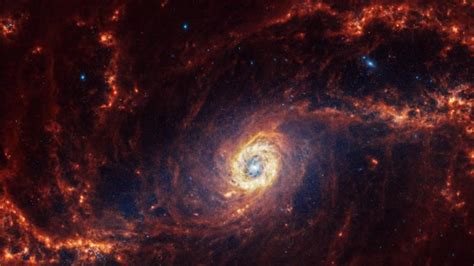
4. Understanding Spooky Action at a Distance
“Spooky action at a distance,” a term coined by Albert Einstein, refers to the perplexing phenomenon of quantum entanglement. This entanglement links particles in such a way that the state of one instantaneously affects the state of another, regardless of the distance separating them. This seemingly “spooky” effect directly contradicts classical ideas of locality and causality, which stipulate that influences must travel through space and time.
In the realm of quantum mechanics, entangled particles are bound together by a shared quantum state. This means their properties are inextricably linked. When a measurement is performed on one entangled particle, its partner’s state is instantly determined, no matter the distance separating them. This extraordinary phenomenon suggests that information regarding the state of one particle is instantaneously communicated to the other, seemingly defying the principle of local realism, a cornerstone of classical physics.
While seemingly paradoxical, entanglement has been rigorously verified through experiments and stands as a fundamental principle in quantum theory. This phenomenon implies that the universe, at its core, functions in ways that challenge our traditional understanding, paving the way for innovative research and technological advancements.
5. Applications of Quantum Entanglement in Technology
Quantum entanglement has emerged as a powerful tool in advancing technology, especially in the fields of communication, computing, and cryptography. One of the most promising applications is quantum cryptography, specifically Quantum Key Distribution (QKD). QKD utilizes entangled particles to establish secure communication channels, ensuring that any attempt to intercept the communication is immediately detected, thus providing unparalleled levels of security.
Quantum computing leverages entanglement to execute intricate calculations at speeds that classical computers cannot match. Entangled qubits, the basic building blocks of quantum information, possess the unique ability to represent and manipulate data in ways that dramatically amplify computational power. This exponential increase in power enables the resolution of problems that remain currently unsolvable.
Quantum teleportation, another application, leverages entanglement to transfer a particle’s quantum state between locations without physically transporting the particle. This holds promise for secure data transmission and the construction of quantum networks.
Beyond these applications, entanglement is also being explored in fields such as quantum sensing and imaging. In these areas, entanglement can significantly enhance the precision of measurements and the resolution of images. As research in this field advances, the applications of quantum entanglement are expected to revolutionize numerous aspects of technology, transforming the way we live and work.
6. Quantum Entanglement and Information Theory
Quantum entanglement profoundly impacts information theory, revolutionizing our understanding of information processing, transmission, and security. In classical information theory, information is conveyed through bits, existing in either a 0 or 1 state. However, quantum information theory utilizes entangled qubits, which can occupy multiple states concurrently. This enables the representation and manipulation of information in ways that are unattainable with classical bits.
The concept of quantum superposition, which empowers quantum computers to process massive datasets at unprecedented speeds compared to classical computers, is rooted in the property of entanglement. Moreover, entanglement facilitates quantum error correction, a critical mechanism for safeguarding the integrity of information in quantum systems, where traditional error correction techniques prove inadequate.
Quantum Key Distribution (QKD) relies heavily on entanglement, a fundamental quantum phenomenon. QKD utilizes entangled particles to establish an unbreakable encryption system. This method exploits the inherent properties of quantum mechanics to instantly detect any eavesdropping attempts, thereby ensuring the secure transmission of information.
With every new insight into quantum entanglement, the field of information theory undergoes a transformation. This profound understanding is paving the way for groundbreaking technologies that will revolutionize communication, computation, and data security in the emerging quantum age.
7. Challenges and Limitations in Quantum Entanglement Research
Quantum entanglement holds immense promise, but its practical realization faces substantial hurdles. A key challenge lies in sustaining entanglement over extended distances. Entangled particles are extraordinarily sensitive to environmental noise, leading to “decoherence,” a process that disrupts the entangled state. This disruption hinders the use of entanglement for practical purposes, such as quantum communication and computing.
Scaling up quantum systems presents a significant hurdle. While scientists have successfully entangled small groups of particles in laboratories, expanding this to a scale suitable for large-scale quantum computing or secure communication networks is a monumental undertaking. The delicate nature of entangled states necessitates extremely precise control and shielding from external interference, a feat that remains challenging outside of meticulously controlled environments.
Moreover, measuring entangled states without interfering with them presents a significant challenge. The very act of measurement in quantum mechanics often changes the state being observed, posing a hurdle for the practical implementation of entanglement in real-world scenarios.
Finally, substantial technological and theoretical obstacles remain before the full potential of quantum entanglement can be realized. Ongoing research and development are crucial to address these challenges and advance the frontiers of this field.
8. Quantum Entanglement in Quantum Computing
Quantum entanglement lies at the heart of quantum computing, driving its exceptional computational power. While classical computing relies on bits, which can only be 0 or 1, quantum computers utilize qubits. Entanglement enables qubits to exist in multiple states simultaneously, a concept known as superposition. This unique property allows quantum computers to process massive amounts of data concurrently, resulting in a dramatic increase in their computational capacity.
Entanglement empowers quantum computers to execute intricate operations via quantum gates. These gates manipulate entangled qubits in manners impossible for classical gates, unlocking the potential to solve problems currently insurmountable for classical computers. This includes tasks like factoring large numbers, optimizing complex systems, and simulating molecular structures.
Furthermore, entanglement plays a crucial role in quantum algorithms such as Shor’s and Grover’s, enabling them to achieve exponential speed-ups compared to their classical counterparts. As quantum computing technology progresses, entanglement will remain indispensable in unlocking novel computational paradigms that have the potential to transform diverse fields, including cryptography and materials science.
9. Future Prospects and Emerging Research Areas
The future of quantum entanglement research is brimming with potential, with several emerging fields poised to reshape science and technology. One of the most thrilling prospects is the development of quantum networks, which harness entanglement to create exceptionally secure communication systems and a global quantum internet. This would allow for instantaneous, secure transmission of information over vast distances, revolutionizing how we share and protect data.
Another key area of research focuses on improving quantum computing capabilities. By increasing the number of entangled qubits, scientists aim to unlock the potential to tackle more complex problems. This advancement could revolutionize fields like drug discovery, artificial intelligence, and climate modeling, leading to significant breakthroughs.
Entanglement is also finding applications in quantum sensing, where it can improve the accuracy of measurements in fields like gravitational wave detection and medical imaging. Simultaneously, theoretical research continues to delve into the fundamental nature of entanglement, investigating its implications for our comprehension of reality and the structure of the universe.
The continued advancement in these research fields positions quantum entanglement as a central element in scientific innovation. This promises an unprecedented landscape of both opportunities and challenges.
tirfblog.com